
The problems of testing 5G: Part 1
This article is from the CW Journal archive.
When CW Journal asked me to write an article on the subject of testing 5G my first thought was "shurely not" or "You must be joking Mr. Maxwell". However, since you're reading this I guess I must have accepted the challenge. What I couldn't manage was to get the entire article into the assigned space, so this is just the first part, setting the scene with a discussion of 5G. The second part, covering testing, will follow in a later issue of the magazine.
Having had a career in mobile communications standards development going back 26 years to the early days of GSM, I remember each new generation feeling like an insurmountable challenge - but we figured it out! As I look ahead to 5G it's no surprise then that once again it feels as though we are at the bottom of a very great mountain and the summit is out of reach and shrouded in mist.
So, what will make testing 5G different to what came before? Will we figure it all out again or are there indicators this time that we might get frostbite or die of exposure during the ascent? To answer that question, it is necessary to define the scope of 5G.
What’s in a name?
Ever since 3G, the cell tower has been keen to get your phone to say it is hooked up to the highest possible single-figure integer followed by ‘G’. 4G isn’t always really 4G: 5G will probably be similar.
Much has been written on this subject and in order to limit this article to my area of expertise I will focus on the radio access network (RAN) aspects and leave the core network and applications areas to others. The meaning of the term 5G itself is part of the problem as it means different things to different groups. The ITU, who are setting the high-level requirements for the next generation of cellular communications, do not define 5G as such but are using the term International Mobile Telecommunications 2020 (IMT-2020). This follows on from their IMT-2000 for the third generation and IMT-Advanced for the fourth generation. To find a definition of 5G we need to go to 3GPP who are developing standards for radio interface technologies (RITs) to be submitted to the ITU for compliance with IMT-2020 requirements.
In October 2016 3GPP took the decision to brand all NR specifications and, surprisingly, all LTE specifications from Release 15 onwards as "5G". This will include the new developments for IMT-2020 submission but also includes the ongoing evolution of LTE. The term 5G has therefore been broadened by 3GPP to mean anything new in LTE (4G) and the expected NR specifications. The justification is that the submissions to ITU will be a package of NR only and LTE + NR, which will also include the new NB-IoT specifications for mMTC. The recent announcement by AT&T of their "5G Evolution" network is an example of what we are likely to see commercially since this new network is a re-branding of their implementation of the latest 4G LTE enhancements.
At this point it is safe to say that 5G has lost specific meaning as it may refer to existing technology that meets some "5G" performance expectations (like 5G Wi-Fi) rather than referring to the narrower understanding of a new RIT specifically developed to meet the IMT-2020 requirements. As for that new RIT, 3GPP has surpassed its previous achievements in the snappily named Universal Mobile Telecommunications System (UMTS) for 3G and Long Term Evolution (LTE) for 4G to come up with "new radio" (NR) for 5G. Like LTE, the NR term is intended as a placeholder until a proper marketing term like WiFi™ or WiGIG™ is defined: however it's probably already too late and the term NR will likely be with us for the next couple of decades, well past being "new".
So what is NR? This fortunately is a much easier question than "what is 5G?" NR is a next generation air interface which comes with an aptly named "next generation" core network known as NGC. The main feature distinguishing NR from previous generations is its scalability, though there is a risk that this will also become its nemesis. The target for NR is to fulfil the requirements of IMT-2020 covering three distinct use cases: enhanced mobile broadband (eMBB), massive machine type communications (mMTC) and ultra reliable low latency communications (URLLC). It should be evident that the air interface requirements to cover such a broad range of use cases are diverse and no previous air interface standard has attempted to cover such a range of bandwidths, data rates, coverage and energy efficiency. LTE has evolved to address early eMBB needs with LTE-Advanced Pro, and mMTC was initially addressed with the MTC feature set and then by the more ambitious Narrowband IoT (NB-IoT). But NR aims to cover all this and much more in one unified air interface standard.
The Exponentially Variable Numerology is the key differentiator between NR and LTE
The scalability that enables NR to address such a wide range of use cases lies in the concept of variable numerology, which is the term used to describe the relationship between the sub carrier spacing and related parameters in an orthogonal frequency division multiplexing (OFDM) system. In LTE, the subcarrier spacing was fixed at 15 kHz (except for the 7.5 kHz variant for MBMS). The subcarrier spacing determines the symbol length which in turn is linked to the usable cell size (determined by the cyclic prefix), the frame structure and the achievable latency for the shortest acknowledged packet transmissions. The capacity of LTE is based on the occupied bandwidth. LTE supports six channel bandwidths from 1.4 MHz to 20 MHz, and further extends single user data rates through aggregation of up to 5 channels for a maximum of 100 MHz instantaneous bandwidth.
Although LTE covers a wide range of usable bandwidths from 1.4 MHz through to today's 100 MHz this is all done with a fixed carrier spacing of 15 kHz which puts constraints on optimizations for cell size, latency and channel bandwidth (both small and large). To improve on LTE, the NR subcarrier spacing is defined as 15n kHz where n can take both negative and positive values. With n = 0, NR for the most part looks very much like LTE, but with larger n, it is possible to create wider channels without resort to aggregation. Wider spaced subcarriers shorten the symbol period which enables higher data rates and lower latency. At the other end of the scale with n < 0 it is possible to emulate the new NB-IoT air interface with its 3.75 kHz subcarrier spacing, corresponding lower data rates and increased coverage and energy efficiency - all appropriate for the mMTC use case. The exponentially variable numerology is the key differentiator between NR and LTE, and between NR and the pre-5G standard being deployed by Verizon in the US and KT in Korea. This pre-5G standard is based on a fixed 5x multiplier of LTE's subcarrier spacing, suitable for eMBB and fixed wireless access use cases.
A second fundamental difference NR brings to the party is the plan to operate into the mmWave frequency bands. Release 15 has within its scope any frequency up to 52.6 GHz although the initial deployment focus is around 28 GHz and 39 GHz. The primary advantage of the mmWave bands is the large amount of spectrum that may be licensed for mobile communications which could be more than ten times what is currently in widespread use below 3 GHz today. This bandwidth offers equivalent growth in data rates and network capacity that are part of the vision for eMBB to meet anticipated growth in market demands.
In the initial release of NR planned for June 2018, the focus is on the eMBB use case although there is some work addressing the low latency aspects of URLLC. The third use case of mMTC is not a current priority due to the recent release of the NB-IoT specification in release 13 which is seen as a big step up from what was possible with GSM-based GPRS and LTE-based MTC.
GET CW JOURNAL ARTICLES STRAIGHT TO YOUR INBOX Subscribe now |

The final key differentiator of NR over LTE lies in the full exploitation of the spatial domain for the purposes of achieving higher efficiency. LTE flirted with this through spatial multiplexing, often described as MIMO, where the existence of more than one radio path (or eigenmode) was exploited to increase peak data rates by a factor of 2 or 4 or 8 depending on configuration. The returns from spatial multiplexing have been mixed, with figures of 20% capacity increase being typical averaged over the network for 2x2 MIMO systems and diminishing returns for 4x4. Further exploitation in the downlink was achieved through 3D MIMO, or elevation beamforming, where the use of antenna arrays at the base station allowed the cell to be split into near and far sectors, or for per-user beamsteering. Again, the benefits from this early spatial technology are somewhat limited partly since they apply in the downlink only. To fully exploit the spatial domain, we need massive MIMO, where the number of antenna elements is considerably greater than was necessary for basic elevation beamforming.
Massive MIMO is a technology that can be applied to LTE as an add-on or to NR which is being designed to take full advantage of the potential. A key point about the feasibility of massive MIMO is the frequency of operation. The size of antenna arrays scales with the wavelength of the carrier frequency, and at 3 GHz, the elements might be spaced at 50 mm (λ/2) making a 64 element 16 x 4 BS array quite large at 750 x 150 mm. This is why at low frequencies, beamsteering is only usable at the base station. Even then, the benefits are promising since the downlink signals are spatially targeted at the UE which is both energy efficient and means there is a considerable reduction of co-channel interference to the adjacent cell. This will improve SINR across the network allowing lower coding rates to be used resulting in higher spectral efficiency and user data rates.
The testing of NR at MMwave Frequencies will look like nothing we have ever seen before
But the full advantage of massive MIMO is only achieved when it can be applied in both the uplink and downlink, and this is where the deployment of NR in the mmWave frequency bands has the most potential. The use of massive MIMO at low frequencies can be seen as an option (for the downlink), but at mmWave frequencies it is less an option and much more of a necessity. The reason for this lies in the Friis equation which predicts the free space path loss. At 30 GHz, the attenuation in the channel is 30 dB (a thousand times) higher than at 1 GHz. With all else remaining equal it is therefore necessary to mitigate this increased loss if the link budget is to be maintained at levels that are necessary to operate mobile networks. The solution to the higher transmission losses is in the use of directional antenna arrays at both ends of the link. Since the antenna element spacing is inversely proportional to the carrier frequency, a 30 GHz antenna array wil be 10x smaller than its 3 GHz equivalent. The result is that at mmWave frequencies it is feasible to implement large antenna arrays at both ends of the link and not just at the base station. That said, for space and power consumption reasons, the UE will still likely use fewer elements than the base station.
The need and ability to use large antenna arrays at mmWave frequencies creates three unavoidable consequences, one very positive and two very negative:
- Positive The signals generated and received by the antenna arrays are highly directional opening opportunities for spatial multiplexing based on beam steering (rather than less reliable Eigenmodes at lower frequency) with the added benefit of reduced co-channel interference between cells
- Negative The system will only operate if the base station and UE antennas are pointing in the right directions
- Negative The use of antenna arrays and the essential need to validate beamsteering performance mean that ALL mmWave testing must be done "over the air" (OTA)
The need to verify the performance of beamsteering using OTA test methods means the testing of NR at mmWave frequencies will look like nothing we have ever seen before. At less than 6 GHz frequencies NR testing will be more conventional. UE testing will primarily be non-spatial conducted while base stations employing active antenna systems (AAS) will have some of their requirements validated OTA.
At < 6 GHz, the main difference between today's conducted LTE testing and NR will be evolutionary in that the frequencies are likely to be higher (3.4 GHz to 5.99 GHz) and the maximum channel bandwidth will rise from 20 MHz to 100 MHz. The width of some NR bands will also be significantly larger than current LTE bands meaning maintaining device performance across the band will be much harder than for the narrower LTE bands.
Part Two of this article will examine the test challenges for NR at mmWave focussing on the UE.
3GPP 3rd Generation Partnership Project
AAS Active antenna systems
CATR Compact Antenna Test Range
eMBB Enhanced mobile broadband
GSM Global System for Mobile Communications, originally Groupe Spécial Mobile
IMT-2020 International Mobile Telecommunications 2020
ITU International Telecommunication Union
LTE Long Term Evolution (aka 4G)
MBMS Multimedia Broadcast Multicast Services
MIMO Multiple Input, Multiple Output
mMTC Massive machine type communications
mmWave Millimetre Wave (length)
NB-IoT Narrow Band Internet of Things
NR New Radio
OFDM Orthogonal frequency division multiplexing
OTA Over The Air
RAN Radio Access Network
RF Radio frequency
RIT Radio Interface Technology
RRM Radio resource management
SNIR Signal to Interfierence and Noise Radio
TRP Total Radiated Power
UE User Equipment
URLLC Ultra reliable low latency communications
Moray Rumney received a BSc in EE from Heriot-Watt University in 1984. He joined Hewlett-Packard that year and remained with the company through the transition to Agilent Technologies in 1999 and Keysight Technologies in 2015. Moray joined ETSI in 1991 and 3GPP in 1999 where he participated in the development of cellular communications standards and conformance test methods. His recent work has focused on radiated test methods for mobile phones. He was editor and major contributor to Agilent’s book “LTE and the Evolution to 4G Wireless”. From 2018 to 2021 Moray represented Keysight at 3GPP RAN plenary who oversee the development of the 5G radio standards. His recent interests include expert witness work, engineering consulting services, and the safety debate around cellular technology including the evolution of test methods to validate that base stations and mobile phones comply with safety regulations.
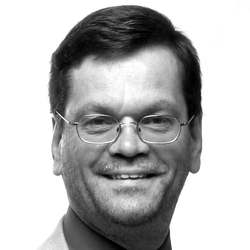