
Reinventing handset radios
Early cellular systems were based on analogue FM mobile radio, had limited capacity, and the handsets were expensive. Fundamentally, humans expect to listen while they talk on the phone, which means that “duplex” operation is needed. The only way to do this with analogue radios is to separate the frequency bands used for transmissions to the handsets from those in which the handsets transmit; and then use a “duplex filter” to share the antenna between the receiver and transmitter while preventing the powerful transmitter signal deafening the receiver – “Frequency Division Duplexing” or FDD. In the early days these filters were bulky and expensive and hand-portables were quite hard to make.
When the Advanced Mobile Phone System (AMPS) system was launched in the USA, two 25MHz wide bands around 800MHz, separated by 45MHz, were allocated. In Europe, an allocation in the 900MHz band enabled similar systems to be deployed, though based on slightly different approaches – an AMPS variant called TACS in the UK and a system called NMT in Scandinavia. This fragmentation wasn’t in customers’ interests, so the European Commission started an activity to standardise a common European cellular system through ETSI which was called GSM1, or now “second generation” or 2G. It was early decided that the system should use digital modulation and coding to best exploit silicon VLSI technology for low cost and high capacity. Since spectrum had already been allocated in duplex tranches across Europe it was natural to use the same frequencies, but a key decision was made to allow handsets to transmit in different timeslots from the ones they received in, so the expensive duplex filter could be replaced with a simple switch. Partly through this decision2, and for many other reasons, GSM was a brilliant technical and commercial success and ignited today’s consumer mobile market.
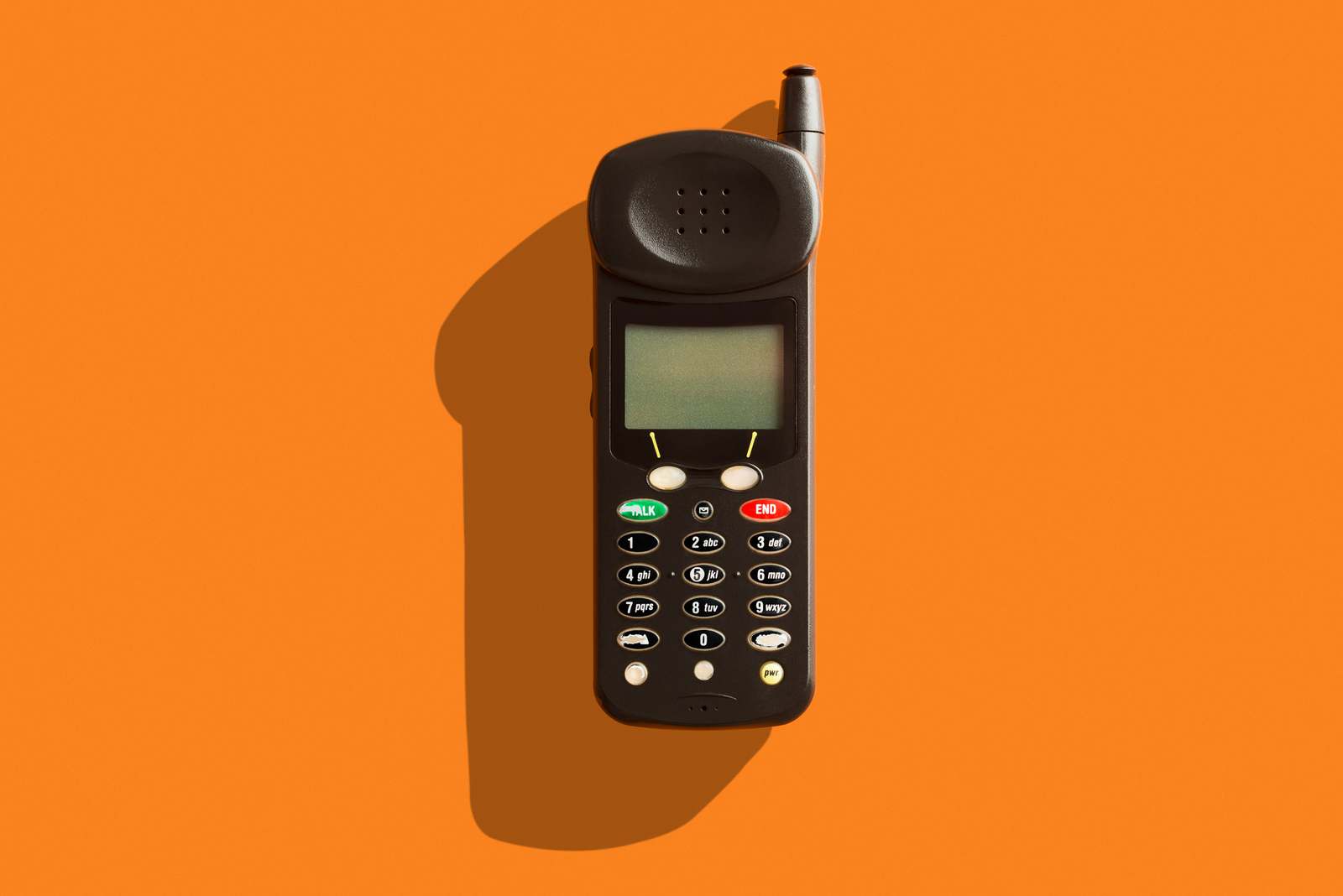
Though it started in the 900 MHz band, GSM was soon being deployed around 1900MHz in Europe, and 800 and 1800MHz in the USA, and in a host of other countries. Soon quad-band handsets were being made that allowed the international traveler to use his phone virtually anywhere in the world; and just as importantly, manufacturers could standardise on a single hardware design for a global market. The phone became a universal consumer good.
It couldn’t last. For a variety of reasons, the industry evolved towards new systems and a global partnership – “3GPP” – brought together regional standards bodies to design a new 3G standard, which could ultimately displace 2G. A combination of commercial, political and technical factors meant that 3G technology operated in the duplex allocations of 2G, but demanded the handsets to transmit and receive at the same time, so duplex filters became a requirement for the new devices3. To start with, since there wasn’t enough spectrum to deploy 3G in the now-congested 2G bands, new bands were allocated, but these tended to be at different frequencies in different regions as regulators struggled to find room. So a “world phone” now needed to have a separate duplex filter for each band, as well as the quad-band 2G radio. And when 3G started to be deployed in the 2G frequencies, each 2G band would need a duplex filter too! As 4G, and now 5G, came along, new bands were allocated but the systems were also expected to work in the existing ones. Today, 3GPP standards define nearly 90 different frequency bands in which equipment can operate, of which well over half are FDD and need a duplex filter4. It has become impracticable to make a single radio that can operate in all bands and be used anywhere in the world – instead the handset vendor has to make a number of band variants aimed at different regions. This makes it harder to gain scale economy, building in additional costs through supporting product variants and needing different approvals. And since the duplex filter is band specific, if new bands are allocated existing equipment cannot make use of them which makes the industry even less sustainable.
The 3GPP bands range in frequency from around 450MHz to nearly 6GHz, the higher frequencies having been allocated for 5G; and the “FR2” bands from 26GHz to 39GHz (also known as “millimetric wave”). Though these new bands potentially have huge capacity the radio can only operate over very short ranges – the “sub-6GHz” bands are overwhelmingly important for coverage of both rural areas (mainly below 1GHz) and in-building, and most sub-GHz spectrum is FDD. Frequency division duplexing is here to stay!
A handset at maximum range needs to receive a signal power 12 orders of magnitude lower than it transmits. In FDD, where the transmitter and receiver operate simultaneously, the duplexer uses filters to isolate the receiver from the high powered transmitter signal. Duplexers in cellular FDD front-ends provide >~50 dB of isolation in the uplink band, to prevent the Tx signal from overloading the receiver, and in the downlink band, to prevent receiver desensitisation due to out-of-band Tx emissions which fall in the Rx band [see Figures (a) and (b)], with minimal loss in the transmit and receive paths.
This low loss and high isolation requirement, at a frequency separation of just a few percent, requires high-Q filtering which can still only be implemented using surface acoustic wave (SAW) or bulk acoustic wave (BAW) devices. Whilst this technology continues to develop, with advances resulting largely from the huge volumes of these devices needed, multi-band operation means a separate off-chip duplexing filter for each band, as shown in figure (c). Increasingly, the PAs, duplexer, and switches are integrated into a front-end module to save space, but this only treats the symptom (hardware size) and not the problem (hardware duplication). All the switches and routing also add extra loss and compromise performance.
(a) Tx and Rx spectra in an FDD system
(b) Duplexing filters to avoid self-interference between the Tx and Rx path
(c) Basic architecture of multi-band FDD transceiver front-end. A separate duplexer is required for each band
The need for small and efficient duplex filters spurred suppliers to develop amazing technologies using various types of piezoelectric resonators to get very high Q factor in small size. Though these enable extremely good filters to be made they have one severe drawback: it is virtually impossible to tune them from one band to another (see boxout). As a result, each band in a handset needs its own duplexer, with associated switches to route the RF signals from the appropriate transceivers for the bands to the antennas. As phones started to use MIMO in downlink and uplink, the number of duplexers multiplied as did the number of switches. All the switches and associated routing add loss, which dissipates transmitted power and makes the receivers less sensitive. As more bands and modes are introduced this complexity and the resulting performance hit increases. The situation has come about because standards design and spectrum policy over the years have failed to consider the cost and performance impact of the resulting complexity. But there is probably no way back when the huge installed infrastructure built around a historical set of spectrum allocations is considered. Instead, a new way to solve the front-end problems is sorely needed.

As explained in the box-out, conventional duplexers depend on RF filters. Filters reject signals either by absorbing or reflecting energy at unwanted frequencies in a given path. For the very first issue of CWJ I wrote an article describing how we can cancel out unwanted signals using a locally generated replica5. Old-fashioned telephone handsets, for example, needed to avoid deafening the user by replaying her own voice in the earpiece while listening to the speaker on the other end of the call. To do this a device called a “hybrid transformer” fed the signal from the microphone to the earpiece in antiphase to the signal applied to the line. In the old days of wireline modems for connecting computer terminals, more complex cancellation was used to allow duplex data communications on the same telephone pair. The same technique can be applied in principle at RF – years ago the Plessey company had a military radio repeater called Groundsat that could be used to extend battlefield communication links. Today there is much interest in this “in-band full duplex” (IBFD) communication method for applications such as Wi-Fi and in defence. In principle using the same spectrum band to communicate simultaneously in both directions could double the capacity of a system and have a huge impact on spectrum efficiency. Though the implementation is formidable it can be done with modern analogue and digital signal processing, and both Wi-Fi and cellular standards bodies are now looking at this technology. Nevertheless it may be some time before IBFD is deployed widely in consumer products, though there are some niche applications on a closer horizon.
After that early article our work at Bristol focused more on applying cancellation to the FDD problem, explicitly to find a way to eliminate the duplex filters. This culminated in a demonstration of a circuit and associated signal processing algorithms that for the first time could meet the relevant 3GPP specifications without using duplex filters in the radio front-end and could be tuned over a wide range of bands from 700 to 2600 MHz6. We combined a hybrid junction with additional RF and digital signal processing and needed only one low-performance tunable filter. This research was pivotal for us as it demonstrated that we could eliminate the duplexer from the handset, bringing in train a number of benefits.
The primary benefit is a reduction in component size for handset manufacturers. In a market where vendors compete aggressively on product features and form-factor, reducing the RF PCB “real estate” makes room for bigger batteries for example. Component cost could be reduced, and a larger number of bands supported without the need for significant component duplication, reducing the need for regional product variants. The technology might eventually bring back the “world phone”.
For users and the industry generally, phone designs will become more flexible. One of the drawbacks of fixed-frequency RF front-ends is that devices are not “future proof”. Operating bands are fixed at the design stage and making use of newly released spectrum means buying a new phone. With a tunable front-end, using new spectrum could just require a software update. The final obstacle to the true “software defined radio” could be removed, eliminating a key technology barrier to extending the product lifetime of a smartphone.
Tunable RF could also enable new ways to license spectrum, such as “Dynamic Spectrum Access” where bands could be shared between services (e.g. cellular, broadcast, emergency services) according to time and location rather than being dedicated to single operators. Studies have shown considerable gains in efficiency are possible.
As the radio becomes more flexible a wider choice and lower cost of devices for obscure frequency bands becomes possible. Indeed the industry may be able to make use of narrower slivers of spectrum that would otherwise not support enough users to make it worthwhile for manufacturers to make phones.
So with all these benefits, how can we implement cancellation duplexing in a mobile handset? Our research showed it could be done and one way to do it, but it was clear that major changes would be needed to both the RF and baseband modem parts of the handset system. A company with control over the whole system could do this, but it would be hard for handset makers to use the technology unless the system components became widely available. Ideally one would have an “integrated front-end module drop-in replacement” for the main parts of a conventional handset that would work with a standard baseband. The problem with the standard filter approach to duplexing is that filters with the selectivity and low loss needed to get enough performance cannot be tuned; while filters that can be tuned and have low enough loss don’t have enough selectivity! What we discovered was a new class of “Adaptive Passive Cancellation” (APC) circuits that combine tunable filtering with cancellation to give the selectivity needed. The cancellation is all done at RF without needing broadband digital signal processing, but the circuit relies on digital processing and adaptive tuning to precisely align the cancelling signal’s amplitude and phase. Forefront RF has practical prototypes working on the bench and is developing miniaturized implementations we can demonstrate with handset manufacturers. We’re currently building out the team needed to take this through to production, planning to sell hardware to phone makers through the fabless semiconductor model.
RF cancellation duplexing has been around for many years and has become a hot topic for academic research. But now with the radio complexity of cellular terminals getting out of control there is a real need for new solutions which is pulling through innovation.
It’s happening now, in Cambridge. Watch this space!
(1) After “Groupe Speciale Mobile”, the initial standards committee name. Later “Global System for Mobile Communications”.
(2) https://www.cambridgewireless.co.uk/news/cw-journal/what-did-gsm-get-right/
(3) Actually 3G includes a time-division duplex mode for some bands but this hasn’t been widely used. 4G and now 5G also have TDD bands in new spectrum but the majority of allocations remain FDD. Handsets therefore need to support both modes!a
(4) Later generations include a TDD mode which doesn’t need a paired frequency allocation (transmitting and receiving on the same frequency alternately), but TDD can’t be deployed in FDD bands and has other shortcomings, so FDD is still a key requirement.
(5) https://www.cambridgewireless.co.uk/news/cw-journal/flexible-duplexing-no-more-self-interference/
(6) Tunable Frequency-Division Duplex RF Front End Using Electrical Balance and Active Cancellation;
Leo Laughlin; Chunqing Zhang; Mark A. Beach; Kevin A. Morris; John L. Haine; Muhammad Kalimuddin Khan; Michael McCullagh; IEEE Transactions on Microwave Theory and Techniques, Volume 66, Issue 12.
Leo’s award-winning research on signal cancellation technology forms the basis of Forefront RF’s product development. He was previously a Research Fellow at the University of Bristol, and has worked in the wireless industry on mobile chipset development. He has a Ph.D in wireless communication, has published in top academic and industry journals, and is named inventor on several patents.
