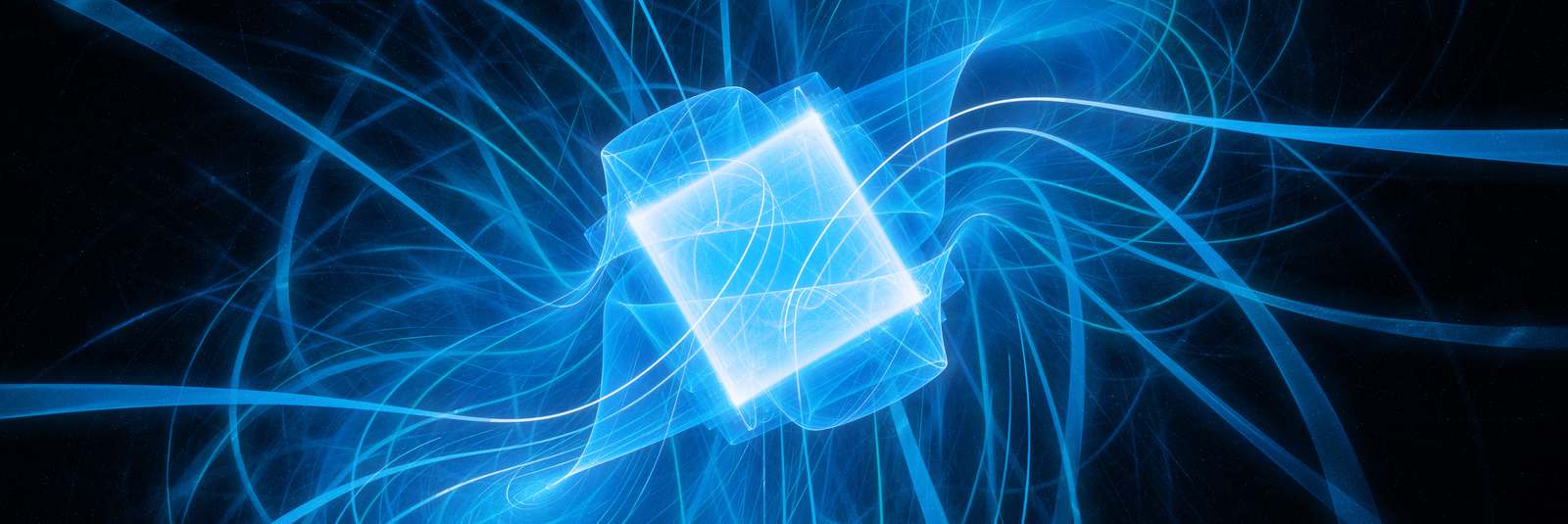
Quantum Technology
This article is from the CW Journal archive.
The single arithmetic logic unit (ALUs), where the work takes place, in today’s computers, are limited to doing one thing at a time, so the more complex the problem, the longer it takes. A really difficult calculation that requires more power and time than a computer can handle is called an ‘intractable’ problem. This is the kind of challenge quantum computers are expected to solve.
"I can navigate the infinite delta streams of future probability and see that there must one day come a computer whose merest operational parameters I am not worthy to calculate, but which it will be my fate eventually to design.” –
Deep Thought computer, The Hitchhiker’s Guide to the Galaxy by Douglas Adams (1978)
Existing digital computers encode data as bits which can be stored in one of two states: 0 or 1. Quantum computers use quantum bits, known as ‘qubits’, that are both 0 and 1 at the same time – and everything in between. Confused? Don’t worry, many quantum physicists find it troubling but they accept it all the same. This counter-intuitive fact, together with the ability of qubits to share a quantum information by ‘entanglement’, allows quantum computers to perform many calculations simultaneously.
The core elements necessary for quantum computers include: a source of qubits, a memory for storing the qubits, quantum logic circuits for executing operations on them, and a means to read the computer’s outputs. The major challenge is in maintaining the quality of the inherently very delicate qubits for long enough to perform quantum computations on them. Qubits quickly lose their special quantum properties – within 100 microseconds for superconducting qubits – due in part to ambient vibrations, temperature fluctuations, and electromagnetic waves. Isolation from this is essential.
Since launching on May 4, 2016, in Milan, users have run more than 10 million quantum experiments on IBM Q, and there have been more than 180,000 downloads of the Qiskit open-source quantum computing framework and more than 150 third-party papers published.
A popular choice has been to encode qubits in micron-sized superconducting loops that exhibit quantum mechanical effects – the so-called superconducting qubits. These show the required ability to store quantum states for a sufficient time and to perform quantum-logic operations. Indeed, D-Wave Systems, a quantum technology company based in Canada, has built and sold a number of quantum-based computing machines based on this idea. Its new Pegasus chip, released in February 2019, has more than 5,000 qubits. While not a truly general-purpose quantum computer – it doesn’t use quantum logic gates – the D-Wave machine is designed to exploit quantum effects to solve optimisation problems with a speed and efficiency that opens up new possibilities.
GET CW JOURNAL ARTICLES STRAIGHT TO YOUR INBOX Subscribe now |
IBM’s Q System One machine, announced in January this year, is a 20-qubit model and is described by the company as the world’s first computer using quantum logic gates. Access is only available via the IBM Cloud because of the computer’s special operational requirements and the company predicts that this will remain the dominant delivery mechanism for the foreseeable future. Customers include ExxonMobil and Cern, which intends to apply quantum machine learning techniques to analyse data from the Large Hadron Collider.
Apart from superconducting loops, another promising idea for qubit arrays is the use of cold, trapped ions or neutral atoms, which offer advantages in terms of qubit stability. IonQ, a US university spinout, aims to encode qubits in single ions held by vacuum traps in electric and magnetic fields, while ColdQuanta, based in the US and the UK, claims to be leading the field in cold atom quantum technology and have their sights on neutral atom qubits.
Microsoft is taking a different approach. It proposes encoding qubits using exotic states of matter called ‘anyons’. These qubits would, in theory, be more resistant to external noise and so make error correction easier. Although anyon qubits have yet to be created, Microsoft is investing heavily in expertise based at the University of Delft in the Netherlands to research the possibilities.
Exotic particles aside, Professor Robert Young, who co-founded a spinout from Lancaster University, called Quantum Base, that specialises in quantum security technology, predicts: “A truly disruptive qubit technology is likely to be based
on silicon”.
Silicon is an attractive platform for qubits because of its compatibility with existing microelectronics circuits and chip manufacturing techniques. As with all qubit proposals, the problem is how the delicate quantum states can be kept alive for long enough to perform quantum operations. In Australia, researchers at the Centre for Quantum Computation and Communication Technology recently produced single-atom qubits embedded in a silicon lattice and controlled using electric fields. These have proven to be relatively robust to electrical noise, allowing them to hold their essential quantum states. By fabricating the chip with a dedicated control layer placed von top of a lower layer of qubits, the 3D architecture aligns its qubits to error-correction control lines – essentially very narrow wires – to constantly correct for errors in many qubits in parallel. Another advantage of this design is that the qubits are very small: literally atom sized. This could allow many qubits to be placed on a small chip, potentially up to two hundred times more than is possible with micron-sized superconducting qubits. Even those in the field, however, admit that silicon-based qubit technologies are playing catch-up with their superconducting qubit competitors.
Quantum computing is now a reality. Many have suggested the biggest remaining challenge is now not the science of quantum computing, but the engineering.
In April 2019, market researcher Tematys presented a European study on quantum technologies. Commissioned by the European Co-operation on Science and Technology (COST) for its Action MP1403 Nanoscale Quantum Optics (NQO) project, the study looked at the market impact of quantum communications and quantum sensing applications.
There is potential in telecoms, online gaming, security, defence, financial services, user/document authentication, and even in ATM withdrawals. The identified key applications are quantum key distribution (QKD) and quantum random number generators (QRNG), this must, however, compete with the well-established and cheaper, True RNG. QRNG aims to generate truly random numbers for use in generating secure cryptographic keys or tokens, while QKD seeks to provide a means for secure exchange of cryptographic keys. A good opportunity for developing a QRNG market is seen by associating it to QKD applications.
NOT HERE YET
The report lists the current stumbling blocks for this technology, in order, as: the need for higher data rate; cost-effective integration; and availability of certification protocols and standards. Surveyed companies said they would be prepared to pay up to an extra 50 per cent for ‘enhanced by QKD’ security.
Tematys foresees development as a three-step market growth: in five years it predicts the deployment of space and airborne QKD technology will produce an overall market of €50m; in seven years, it should be the turn of the ground telecom professional segment to take this up to €150M; and in 10 years the general telecom network will return a market of €300m. To fulfil these estimates, a decrease to at least a fifth of current prices is needed.
Quantum systems are fragile but quantum sensing turns this weakness into a strength to measure properties at precisions never achieved before. The report focused on sensing systems such as nitrogen vacancies (NV) defects in diamonds and atoms. Atomic sensors find applications in oil and gas explorations, satellite telecommunications, seismology, the study of ocean dynamics, global navigation, volcanology, and fundamental physics.
Atomic sensors, based on the optical properties of laser-cooled ions and atoms, include optical atomic clocks (OACs) and matter-wave interferometers (MWIs). In the last eight years, OACs have undergone constant improvements, achieving a record relative precision of 2.5×10-19. Weightlessness in space would eliminate restraints due to gravity, but OAC systems would have to be simplified in some way, such as integrated photonics.
Generation in space of quantum states, key to MWIs, have been demonstrated by China, Germany and the USA. MWIs exploit the fact that laser-cooled atoms, close to absolute zero, begin to act like matter waves, making devices ideal as accelerometers or gravity sensors.
Nitrogen-vacancy centres, which are incredibly sensitive to magnetic fields are able to detect a single spin with nanometre spatial precision and proximity. Being biocompatible, prospective applications are in life sciences (magnetic cell-labelling), in magnetic recording (imaging a hard drive’s read-head field), or in measuring tiny currents.
The COST-sponsored report surveyed preferences for nanoscale quantum sensing. Measuring magnetic fields was the top requirement, followed by time and temperature. Key applications were fundamental research, followed by biomedicine and geophysics. The market growth forecast for atomic-chip sensors shows steady growth, with a market size up to €15m in five years, but for NV centres all manufacturers predict ‘rocketing’ growth, reaching a market of up to €60m in the same timespan. For the next five years atomic clocks will still represent the largest market for quantum communication and sensing. Within the next 10 years, the related telecom plus the sensing and imaging, markets should become close competitors. It is predicted that, overall, the accumulated 10-year market will be of the order of €7bn, with a tenfold market in associated services and systems.
The free study is available at www.cost-nqo.eu/support/documents/
Irene D’Amico, University of York
Quantum security refers to communications that use QKD (Quantum Key Distribution) and QRBG (Quantum Random Bit Generation) to securely transmit data. Networks up to 100km employing QKD are being developed and repeaters aim to support longer-distance distribution of cryptographic keys through such networks.
In the near-term, quantum random number generators and point-to-point quantum key distribution are at, or close to being, market-ready. QKD networks and the necessary quantum repeaters are mid-term to long-term technologies in terms of readiness.
The inherently random nature of quantum mechanics, means QRNG, unlike other technologies, generates truly random numbers resulting in stronger cryptographic keys. Commercially available QRNG systems are able to provide up to 1 Gbps outputs, demonstrating technology compatible with commercial servers and certified to national standards in several countries.
Quantum key distribution exploits quantum mechanics. It allows two parties to share a cryptographic key with the advantage of being able to detect if a third-party eavesdropper is intercepting communications. QKD in its most common form uses individual photons to convey the separate bits of a cryptographic key. QKD systems using fibre-optics are currently able to operate over standard telecoms fibre links, either as dedicated fibres or as fibres carrying other traffic. However, transmission through optical fibres is limited by losses that increase rapidly with distance. For example, single photons sent natively over 1,000km of optical fibre at rates of 10GHz, would require a receiver to wait over a hundred years to detect just one. This has limited the range of QKD to distances just over a hundred kilometres meaning that repeaters are needed to extend the range.
Existing telecommunication solves the problem of optical fibre losses by using amplifiers along a fibre to boost the signal. Unfortunately, these can’t be used in QKD networks because they would destroy the quantum characteristics of the photons. This isn’t helped by the fact that quantum physics precludes the copying of quantum information – so simply reproducing an amplified copy of the quantum data is impossible.
A solution to this is to use the weird properties of ‘entanglement’. A photon in an entangled pair is able to mutually share information (its state) with another, effectively replicating itself, even when separated over great distances. A quantum repeater, as with existing non-quantum repeaters, breaks-up the transmission length into lower-loss segments. A photon reaching the end of a segment influences a photon at the beginning of the next segment to create an entangled twin instantaneously. This allows quantum information to be preserved and, effectively, teleported into the next segment of the fibre-optical path for onward transmission. To achieve this a quantum memory is needed and a photon received by the quantum memory of one segment of the transmission line is not measured and destroyed but stored while the next transmission segment is made ready to receive and replicate the quantum information.
A critical element for this long-distance QKD scheme is to create quantum memories for storing photon qubits. These memories need to be able to store qubits reliably for durations long enough to satisfy network transmission times – this means about 100ms for a global communication network. While they are not yet commercially available, they are being developed in a wide range of technology platforms such as ensembles of trapped atoms or ions or using solid-state crystals. Research at Australia’s Centre for Quantum Computation and Communication Technology has dramatically improved the storage time of a quantum memory. It can also operate in the same optical wavelength as current telecommunications infrastructure, making it compatible with existing fibre-optic cables. Using erbium ions in a crystal, the researchers have been able to store quantum information for more than a second – a huge 10,000 times longer than other attempts and brings a global quantum network a step closer.
Nevertheless, there is also a workaround called free-space QKD at hand. Links to satellites have been developed because optical losses for free-space transmissions increase much less rapidly with distance than fibre-optic cables. The Chinese government launched the Quantum Experiments at Space Scale (QUESS) satellite in 2016 and demonstrated QKD data transmission rates of several tens of kilohertz from low-Earth orbit to ground stations, requiring a relatively modest one metre aperture receiver telescope.
The QUESS satellite has created a QKD channel between Vienna and China, establishing the first secure intercontinental quantum video call. While the QUESS system has its limitations – it cannot operate in sunlight and needs line-of-sight to communicate with a ground station – this is, without doubt, an impressive achievement. Canada, Singapore, Japan and several European states each now have their own, or joint, satellite QKD project for low-Earth, medium-Earth, and even geostationary orbits.
Civil and military operations already base many strategic services on satellite communications and QKD may, in future, offer a much greater level of security for ground-to-satellite communications. This opens the possibility of a QKD satellite constellation offering high availability, secure communications between remote ground stations.
Core to QKD systems is the ability to provide single, identical photons for encoding reliably and on-demand, and to subsequently detect them accurately for decoding. One way to provide single photons is to attenuate regular laser pulses, containing many photons, to a single photon in each pulse. However, a growing opinion is that laser-based single-photon sources are a bottleneck to development. This has led to an industry focus on developing so-called ‘quantum emitter’ sources which generate photons one at a time. Much work has been done in this regard using nanophotonic techniques employing, for example, quantum dots, colour centres in diamonds, or photonic crystals to achieve on-chip quantum emitters capable of photon count rates exceeding several tens of megahertz. Single-photon avalanche photodiodes (SPAD) and super-conducting single-photon detectors (SSPD) also currently provide a platform for detection from the ultra-violet to the mid-infrared spectral range, with dead-times as low as a few nanoseconds and ‘dark-counts’ (false detection noise) below one hertz.
In spite of these impressive developments, the widely held view is that data rates, cost-effective integration, and certification need to improve before QKD technologies will be widely deployed. This motivates many of those aiming to disrupt the telecommunications field with new quantum technologies. Robert Young of Quantum Base explains that the potential of QKD should not be limited to customers with deep pockets, so a focus should include developing scalable, practical products: “The promise of quantum communications is incredibly exciting, as it represents a new means to share information that is fundamentally secure.”
GET INVOLVED WITH THE CW JOURNAL & OTHER CW ACTIVITIES |
Quantum sensing covers motion – including acceleration, rotation, and gravity; electric and magnetic fields; and imaging. With such fundamental measurements there are many possible application areas, including aerospace and transport, civil engineering and medicine.
Sensing will benefit from the Government’s UK National Quantum Technologies Programme (NQTP) fund of £400m. with investment in the National Quantum Technology Hub in Sensors and Metrology led by Birmingham University and the Quantic hub led by Glasgow University, together with the significant tranche of £70m for the Industrial Strategy Challenge Fund. To see a return on this investment the Government will need to ensure that the export path for industries is as efficient as possible.
Many of the different quantum technologies can be used in diverse areas. For example, cold atom technologies, which typically use superposition, are used for sensing applications. Cross-fertilisations like this have promoted the rapid development of technologies.
Cold atom technologies involve cooling atoms in a vacuum chamber to a few microkelvins above absolute zero. In this state, the atoms are sensitive to motion, particularly acceleration (gravity) and rotation. Gravity sensors based on this are able to provide a view underground on brownfield building sites and roads, allowing faster survey times with decreased operational overheads. They can also be used in airborne applications for mineral exploration.
Integrating acceleration and rotation sensors creates inertial measurement systems with more than two orders of magnitude improvement over today’s implementations. There is also the possibility of developing inertial navigation systems that could reduce reliance on GNSS satellites. Several Innovate UK projects are investigating this area and will soon publish the results and detail the next steps.
Quantum imaging sensors have also been developed that can detect single photons, allowing range gating with impressive timing accuracy. Applications include collision avoidance and low-energy medical imaging.
Another development has been the use of quantum principles in magnetic field sensing. Both cold atom and nitrogen vacancy (NV) in diamond have been used for this purpose. NV is an important technology as it works at room temperature and, apart from improving magnetometers, it is already showing promise as a way to improve magnetic resonance imaging (MRI) scanners, particularly for examining the brain and heart, by reducing the magnetic field created. NV also paves the way to decreased size, weight, power and cost (SWaP-C) of MRIs compared with equivalent scanning technologies.
One of the benefits of quantum sensing is the improvement in sensitivity over classical systems. The future for quantum sensing is promising and the renewed government investment and increasing interest from industry provide the UK with the capability to continue as a world leader.
Atomic clocks date back to the mid-1950s when Louis Essen developed the first caesium atomic clock at the UK’s National Physical Laboratory (NPL). Accuracy has since improved by six orders of magnitude to around 10-16.
Since the CW seminar on quantum technologies, the US stock market “flash crash” has led to an increase in the requirement for time accuracy. The response has been new types of clock and the development of the chip scale atomic clock (CSAC). With a two orders of magnitude reduction in size, weight and power, it has led to new applications. These have brought an increased focus on the accuracy and reliability of timing in critical applications, ranging from mobile phone infrastructure and national power grid to financial trading.
There has been an increase in the need for clocks of different form, function and cost to different market applications.
Clocks can be split into three different classes: national time standards, global navigation satellite system (GNSS) replacement, and measurement synchronisation.
Aimed at fractional uncertainties of 1 part in 1018 or below. The inner vacuum chamber of the next generation single-ion Yb+ clock from the PTB lab. The ion trap is mounted on a thick copper electrode coming from the top.
Development of national time standards pushes the boundaries of physics to create new clocks capable of increased accuracy. A variety of clocks are under international consideration. Optical clocks are the current state of the art with demonstrated accuracies in the region of 10-18. These are developed by the national measurement institutions and adopted when reliability and accuracy have been proven.
The vulnerability of GNSS signals to accident, deliberate jamming and spoofing, together with the reliance on timing signals is resulting in a reconsideration of their reliability. Implementors must decide whether the free-running time is adequate or if an alternative traceable clock reference is needed (as can be found in financial trading). QT clock designs are being created for commercial markets. For example, an original clock design by NPL is in the process of being commercialised by Teledyne e2v as the Minac miniature atomic clock. NPL also has other clock designs under development.
Atomic clocks provide capabilities in the synchronisation of measurements. One example is the Square Kilometre Array radio telescope with a proposed measurement synchronisation to femtoseconds at each antenna.
The advent of the CSAC has also led to the possibility of using synchronised measurements from sensors placed on multiple lightweight and mobile platforms, such as drones. In the synchronisation of 5G, increased density of base stations may need better clocks.
Accurate timing sources are also accurate frequency references. The greater the accuracy of the source, the lower the noise of the frequency reference. QT has the potential to offer levels of phase noise which will be truly disruptive. Optical clocks, for example, have significant potential to improve performance in radar and other signal detection systems.
One challenge is the development of technologies to produce a compact version of an optical frequency comb to convert a precision optical signal to RF. Various projects around the world have focused on this and, in the UK, NPL is working on a microresonator-based comb to measure and read-out the frequencies of optical clocks. Timing and frequency references underpin vital systems that we rely on every day and increasing their accuracy also allows us to see further out into the universe. References based on quantum principles have been in use for decades but the need for size, weight and power improvements are always there. Developments using a range of different quantum properties are underway which aim to meet these new requirements.
Paul Martin, PA Consulting
The skills required to support the QT industry can be split into three categories: specific QT skills, design and manufacture of technology, and commercialisation of new technology. Specific QT skills are supported through a number of avenues, helped by the popularity of QT subjects at both undergraduate and postgraduate levels. There are three centres for doctoral training (CDTs) specifically for QT which have been in place for phase one of the NQTP and are now in the process of being renewed.
Design and manufacture of technology requires skills at a variety of levels but not necessarily specific to QT. Skill levels range from experienced manufacturing engineers to software experts (including embedded experts) and technicians to build, test and evolve the designs of the complex quantum technologies. The types of skills required need good knowledge and qualifications at different levels in Stem subjects – and these are already in short supply.
The third required skill set is the ability to take a new technology and bring it to commercial success. Further skills are required in solving the commercial issues of making a new technology available and desirable, such as supply-chain creation, business case development and warranties.
Training in QT for scientists, engineers and wider commercial staff is vital to ensure rapid take up. A good start has been made in creating knowledgeable QT experts at the postgraduate level. This now needs to be extended, together with an additional focus on increasing the innovative and commercial skills.
GET CW JOURNAL ARTICLES STRAIGHT TO YOUR INBOX Subscribe now |
Andrew is a patent attorney (Senior Associate) in the Electronics & Software team at Mewburn Ellis LLP. He has a particular interest in telecommunications, photonics, radar, and Quantum Technologies having spent time as a research physicist at the Max Planck Institute in Munich. Andrew handles patent portfolios in this field for global corporations and for a leading university research departments, and also handles subject matter in the defence, electronics, software and general engineering fields. Andrew also has an interest in tecnologies involving the application of computational physics and simulation, and works with clients innovating in the field of Bioinformatics for drug discovery using techniques ranging from neural networks to molecular modelling and classification/regression models.
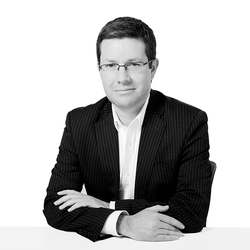